G18CrMo2 ⁃ 6 steel is a typical low alloy Cr ⁃ Mo steel, widely used in the manufacturing of nuclear power turbine cylinder castings. Due to its harsh working conditions, it is required to have high strength, good plasticity, and toughness during service. However, in the actual service process, there is often a mismatch between strength and toughness, that is, when the strength meets the requirements for use, the toughness is poor and often fluctuates greatly. Research has shown that the mechanical properties of low alloy Cr ⁃ Mo steel are closely related to its microstructure. Therefore, studying the heat treatment process of G18CrMo2 ⁃ 6 steel has important research value for improving the mechanical properties of G18CrMo2 ⁃ 6 steel.
Researchers have conducted extensive research on the microstructure and mechanical properties of Cr ⁃ Mo and Cr ⁃ V steels after normalizing and tempering. They have found that martensite austenite (M ⁃ A) islands in the microstructure have a significant impact on toughness. It is believed that the brittle and hard martensite (M) in M ⁃ A islands will deteriorate the low-temperature impact performance of low alloy steels, This is because M in the M ⁃ A island has a higher C concentration and hardness than the bainitic ferrite matrix. Wang et al. believed that the strength of the material increased with the increase of volume fraction of Flat noodles bainite (LB) and M ⁃ A island, while the increase of yield strength was related to the volume fraction of bainite, not to the volume fraction of M ⁃ A island. Li et al. believed that with the extension of annealing time, the proportion of M ⁃⁃⁃⁃⁃⁃⁃ A islands gradually decreased, and the morphology gradually evolved from large block or island to fine particle or strip. This is because the grain growth of austenite and the uniform distribution of Mn reduce the thermal stability of austenite, thereby increasing the volume fraction of tempered martensite (TM) and effectively refining the microstructure. Dong et al. believe that residual austenite or M ⁃ A islands with large volume fraction, mixed morphology of flakes and blocks ensure the stability and persistence of the TRIP effect. Ramachandran et al. found that as the cooling rate changes from low to high, the mixed structure of acicular ferrite (AF) and bainite ferrite (BF) transforms into a mixed structure of polygonal ferrite (PF), granular bainite (GB), and M ⁃ A island. The mixed structure of AF and BF provides better toughness, while PF The mixed structure of GB and M ⁃ A islands makes the sample more brittle. This article studies the effect of normalizing temperature on the microstructure and mechanical properties of G18CrMo2 ⁃ 6 steel, determines the appropriate normalizing heating temperature, and provides a basis for determining the normalizing austenitization temperature of G18CrMo2 ⁃ 6 steel in actual manufacturing.
1. Test materials and methods
The experimental steel is G18CrMo2 ⁃ 6 heat-resistant steel, which is melted and cast into 25kg steel ingots using a vacuum smelting furnace. Its chemical composition is shown in Table 1.

Firstly, cut the casting into 70mm x 48mm x 12mm test blocks using a wire cutting machine, and heat them up to 850, 880, 910, 940, 970, and 1000 ℃ for 2 hours. Finally, cool the samples to room temperature and cut metallographic samples from the heat-treated test blocks. After grinding and polishing, corrode them with 4% nitric acid alcohol for 10 seconds, Observation was conducted using JSM ⁃ 6510 scanning electron microscopy (SEM). Additionally, M10 tensile specimens and V-notch impact specimens were cut from the heat-treated specimens, and mechanical properties were tested using the Z050 universal testing machine and the ZwickRKP ⁃ 450 impact testing machine.
2. Experimental results and analysis
1. The influence of normalizing temperature on microstructure
Figure 1 shows the microstructure of G18CrMo2 ⁃ 6 steel after normalizing at different temperatures. It can be seen that although the normalizing temperatures are different, their microstructures are all granular bainite structures, composed of bainite ferrite (BF) and M ⁃ A islands. The number, size, and bainite ferrite size of M ⁃ A islands were statistically analyzed, and the results are shown in Table 2. As the normalizing temperature increases, the number and size of M ⁃ A islands show a trend of first decreasing and then increasing, while the grain size of bainite ferrite does not change much between 850 and 940 ℃, about 22 μ m. Between 970-1000 ℃, the grain size is significantly larger, reaching 54.9% μ M. The size, quantity, and grain size of M-A islands are closely related to the normalizing temperature.
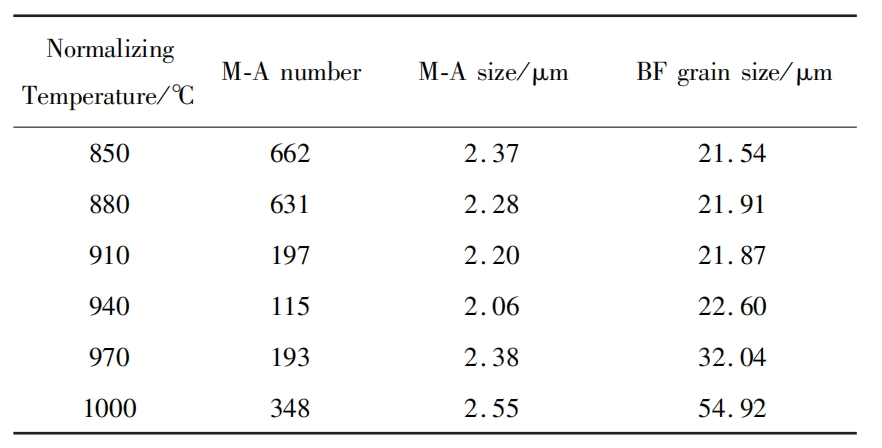
The formation of M ⁃ A islands is due to the bainitic phase transformation during air cooling, where carbon atoms diffuse from the ferrite/austenite interface into the austenite, increasing the carbon content of austenite and stabilizing it. Subsequently, during the cooling process, some carbon rich austenite undergoes martensitic transformation, forming M ⁃ A islands with stabilized austenite. According to Figure 1 (a), it can be seen that at a normalizing temperature of 850 ℃, austenite preferentially forms at the grain boundaries of ferrite. Due to the lower normalizing temperature at this time, which is between Ac1 and Ac3, some of the ferrite inside the grains cannot be transformed into austenite and will remain in the structure. Similar reports have been made in the research of Shi Lihui et al. The presence of ferrite leads to an increase in the content of stable elements (such as C) in austenite, which increases the stability of austenite. During the air cooling process, more austenite is retained, resulting in larger and more numerous M ⁃ A islands. When normalizing at 880 ℃, the diffusion of elements accelerates, the content of ferrite in the tissue decreases compared to when normalizing at 850 ℃, and the number and size of M ⁃ A islands formed during the cooling process decrease, as shown in Figure 1 (b).
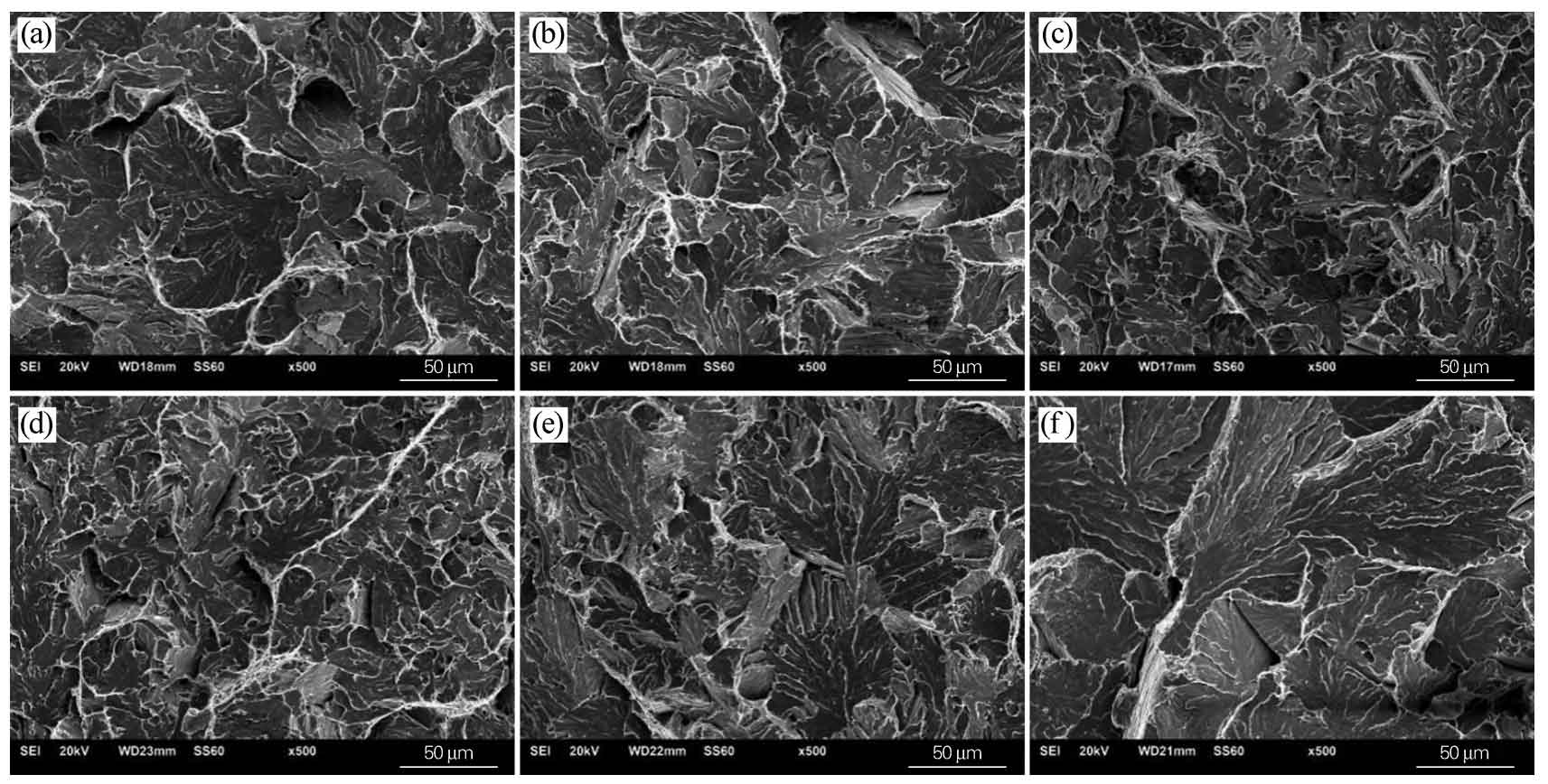
According to Figure 1 (c, d), it can be seen that when normalized at 910 ℃ and 940 ℃, the number and size of M ⁃ A islands in the microstructure are significantly reduced compared to those normalized at 850 ℃ and 880 ℃. Due to the normalizing temperature being above Ac3, the structure is completely austenitized. The increase in normalizing temperature increases the diffusion driving force of alloy elements, improves the uniformity of alloy elements inside the grains, and there is no untransformed ferrite, resulting in a decrease in austenite stability. During the cooling process, the number of M ⁃ A islands sharply decreases and the size decreases.
As shown in Figure 1 (e), the number and size of M ⁃ A islands in the microstructure after normalizing at 970 ℃ have increased. This is because further increasing the normalizing temperature leads to the growth of austenite grains, especially when the austenitization temperature rises from 970 ℃ to 1000 ℃, the increase in grain size is significant. This is due to the decrease in the overall interface energy of the system, which leads to the growth of austenite grains, manifested by the migration of grain boundaries. Atoms are more easily diffused near the grain boundaries, resulting in a decrease in the uniformity of elements inside the grains. At the same time, the number of crystal defects contained in the austenite grains decreases, the rate of undercooled austenite forming bainite decreases, and the position of bainite transformation nucleation decreases. Therefore, the bainite transformation is inhibited. Grain growth and bainite transformation are inhibited, which relatively increases the stability of austenite and forms more M ⁃ A islands. The higher the normalizing temperature, the coarser the grain size, and the faster the element diffusion. During the cooling process, larger and more numerous M ⁃ A islands are formed, as shown in Figure 1 (f).
2. The influence of normalizing temperature on mechanical properties
The mechanical properties of G18CrMo2 ⁃ 6 steel after normalizing at different temperatures are shown in Figure 2. It can be seen that as the normalizing temperature increases, the yield strength and tensile strength show a trend of first increasing and then decreasing, as shown in Figure 2 (a). When the normalizing temperature is 940 ℃, the tensile strength is highest at 942MPa. When the normalizing temperature rises to 1000 ℃, the tensile strength is lowest at 851MPa. As the normalizing temperature increases, the overall impact absorption energy also shows a trend of first increasing and then decreasing, as shown in Figure 2 (b). When the normalizing temperature is 940 ℃, the impact absorption energy is highest at 28J. When the normalizing temperature increases to 970 ℃, the impact absorption energy rapidly decreases. Further increasing the normalizing temperature results in a slow decrease in impact absorption energy. At 1000 ℃, the impact absorption energy is lowest at 11J.
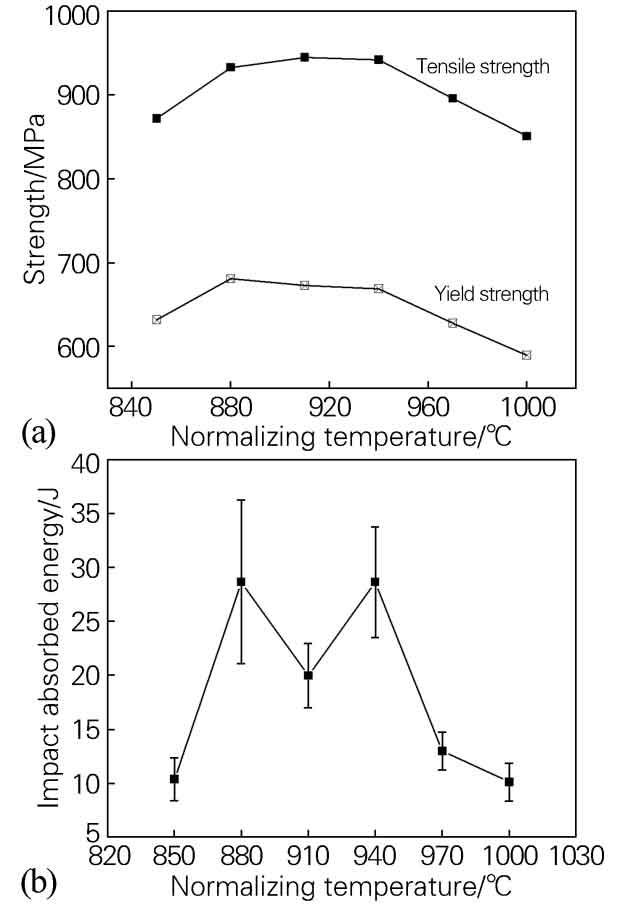
The impact fracture morphology of G18CrMo2 ⁃ 6 steel after normalizing at different temperatures is shown in Figure 3. It can be seen that the fractures at different normalizing temperatures are all cleavage fractures or quasi cleavage fractures. The size of small facets on the cleavage surface varies at different temperatures. As the normalizing temperature increases, the size of small facets first decreases and then increases. When normalizing at 940 ℃, the area of small facets on the cleavage surface is the smallest, indicating that the crack propagation path is short and the propagation direction of microcracks needs more energy to change after being blocked. Therefore, the impact absorption energy is highest at a normalizing temperature of 940 ℃. As the normalizing temperature further increases, the area of cleavage facets increases. After normalizing at 1000 ℃, the cleavage small facet area is the largest, and the energy consumed during microcrack propagation is less. The impact absorption energy is lower, which is consistent with the trend of impact performance changes in Figure 2 (b).
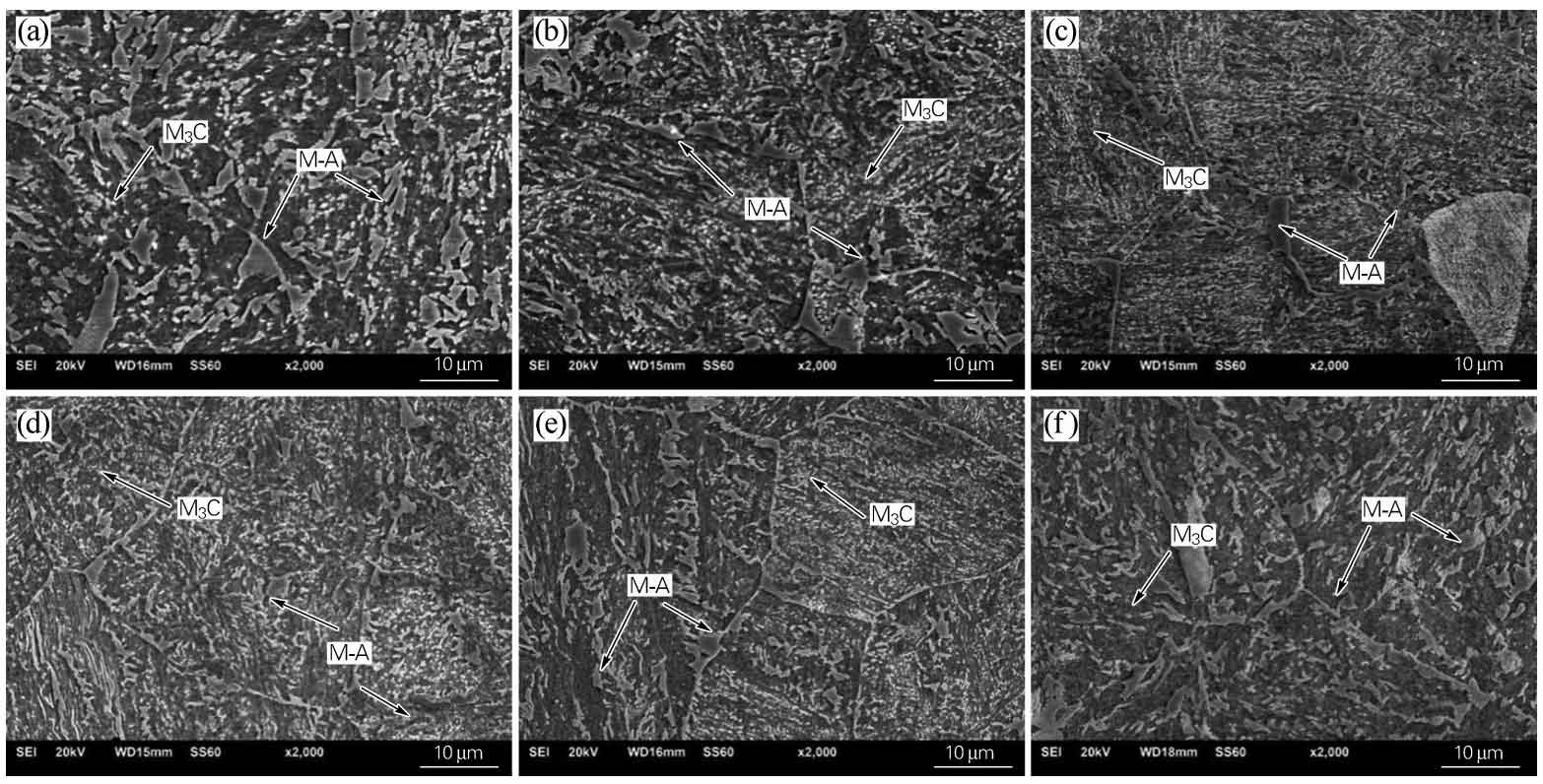
Related studies have shown that due to the hardness of the M ⁃ A island compared to the surrounding bainitic ferrite matrix, it can lead to local embrittlement. When the matrix is subjected to external stress, due to the different deformation abilities of M ⁃ A island and BF, and the poor deformation ability of M ⁃ A island, stress is easily concentrated on M ⁃ A island, resulting in microcracks. The toughness of steel is closely related to the number and size of M ⁃ A islands. When the normalizing temperature is between Ac1 and Ac3, the microstructure containing BF and large-sized M ⁃ A islands is more likely to cause stress concentration. The larger the size of the crack source, the easier the crack is to propagate, resulting in poorer impact performance. When the normalizing temperatures are 910 ℃ and 940 ℃, the microstructure is completely austenitized, the number of M ⁃ A islands is reduced, stress concentration is alleviated, and impact performance is improved. Further increasing the normalizing temperature increases the grain size of the original austenite, reduces toughness and plasticity, and increases the tendency for cracking. At the same time, the number of M ⁃ A islands inside the grains shows an increasing trend, and an increase in the number of M ⁃ A islands will deteriorate the impact performance, resulting in poor impact performance.
As the normalizing temperature increases, the yield strength of the test steel shows a trend of first increasing and then decreasing, which is related to the strength of bainite ferrite and grain size. During the formation of granular bainite, the ferrite precipitated from undercooled austenite is lath shaped with high dislocation density. Compared with ferrite, granular bainite has finer ferrite Flat noodles and higher dislocation density. At the same time, the volume fraction of M ⁃ A island decreases, resulting in the diffusion of C, Cr and other elements in M ⁃ A island to the bainite ferrite matrix, resulting in an increase in the yield strength of bainite ferrite. When the normalizing temperatures are 850 ℃ and 880 ℃, the size and quantity of M ⁃ A islands are larger, and the alloy element content in the bainite is lower, resulting in lower matrix strength. As the normalizing temperature increases, when the normalizing temperatures are 910 ℃ and 940 ℃, the size and quantity of M ⁃ A islands decrease, the alloy element content in the bainitic ferrite matrix increases, and the strength of the matrix improves. When the normalizing temperature is further increased to 970 ℃ and 1000 ℃, the defects in the bainitic ferrite matrix decrease and the grain size increases; At the same time, the number of M ⁃ A islands in the organization increases, and the element content in the bainitic ferrite matrix decreases. The above reasons lead to a decrease in yield strength.
Based on the above analysis, the mechanical properties of G18CrMo2 ⁃ 6 steel are excellent when normalized at 940 ℃. At this time, the number of M ⁃ A islands in the microstructure is the least, and most of them are distributed at grain boundaries. The carbides in the bainitic ferrite matrix are evenly distributed.
3. conclusion
1) After normalizing at 850-1000 ℃, the microstructure of G18CrMo2 ⁃ 6 steel is composed of bainite ferrite and M ⁃ A islands. As the normalizing temperature increases, the number and size of M ⁃ A islands in granular bainite first decrease and then increase. At the same time, the grain size of bainite ferrite does not change much between 850-940 ℃, but significantly increases between 940-1000 ℃.
2) The impact performance of G18CrMo2 ⁃ 6 steel shows a trend of first increasing and then decreasing with the increase of normalizing temperature, which is corresponding to the trend of first decreasing and then increasing in the number and size of M ⁃ A islands with the increase of normalizing temperature. The main reason affecting the impact performance of G18CrMo2 ⁃ 6 steel is the size and quantity of M ⁃ A islands. The yield strength also shows a trend of first increasing and then decreasing with the increase of normalizing temperature. This is because as the normalizing temperature increases, the number and size of M ⁃ A islands decrease, leading to an increase in the content of alloying elements in bainitic ferrite and an increase in yield strength. When the normalizing temperature is 940 ℃, the tensile strength and impact absorption energy are the highest, at 942MPa and 28J, respectively. As the normalizing temperature further increases, the number of dislocations in the bainitic ferrite decreases, the grain size becomes coarse, and the content of alloying elements decreases, resulting in a decrease in yield strength.